NUS Presidential Young Professor Alvin Chua, a faculty at NUS Physics, shares with Physics Matters his research interests and works in the field of astrophysics.
Share with us your current research interests and works in the field of astrophysics.
The setting for most of my research is the field of gravitational-wave astronomy, which involves the study of astrophysical phenomena through their gravitational radiation. While the theoretical prediction and experimental verification of gravitational waves both took place in the previous century, the harnessing of such waves for astronomy is a far more recent development, made possible only by the most sensitive interferometers ever built.
Since gravitational-wave astronomy is barely out of its infancy (as subdisciplines of physics go), it contains more than its fair share of challenges and open problems. As a theorist in the field, I apply modern computational and statistical techniques to the two-sided scientific problem of source modelling (describing astrophysical sources using general relativity) and data analysis (extracting and measuring signals in noisy detector data). My recent focus is on binaries with extreme mass ratios (1:10000 or more), which will be important sources for near-future space-based detectors. I also dabble broadly in machine learning for science, as well as more fundamental topics in applied and computational statistics.
What to you is one fascinating discovery of our universe in recent history?
The very existence of gravitational-wave astronomy is founded on one such discovery, of course—the historic first direct detection of gravitational waves by the LIGO detectors back in 2015 (and, to slightly less public fanfare, the first multi-messenger observation of a neutron-star binary merger in 2017).
With that obvious answer out of the way, I also think the successes of the present Mars-rover missions are some of the most fascinating scientific developments in recent years. These are not discoveries per se, but NASA’s Perseverance (along with its flying companion, Ingenuity) and CNSA’s Zhurong are exploring our planetary neighbour in many unprecedented ways right now, and are vastly improving our understanding of its environment. With a lot of luck and planning, scientists on Earth might even get their actual hands on Martian samples one day.
While the reality of space exploration has never in its history been separable from the perverse spectacle of nationalistic or capitalist “space races”, I do think its spirit remains one of humankind’s most far-sighted and forward-thinking ideals. To me that spirit is ultimately incorruptible, and is worth preserving even (or perhaps especially) in our troubled world.
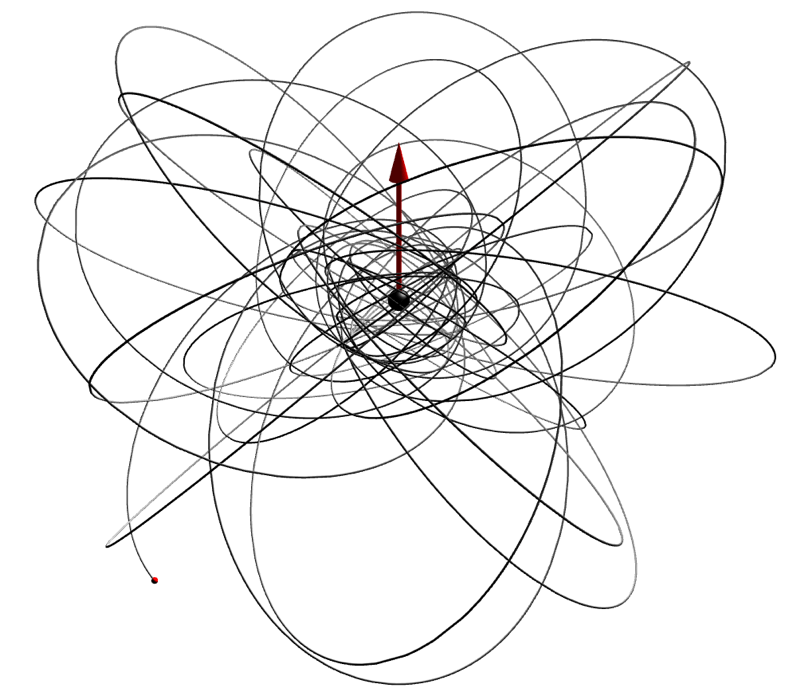
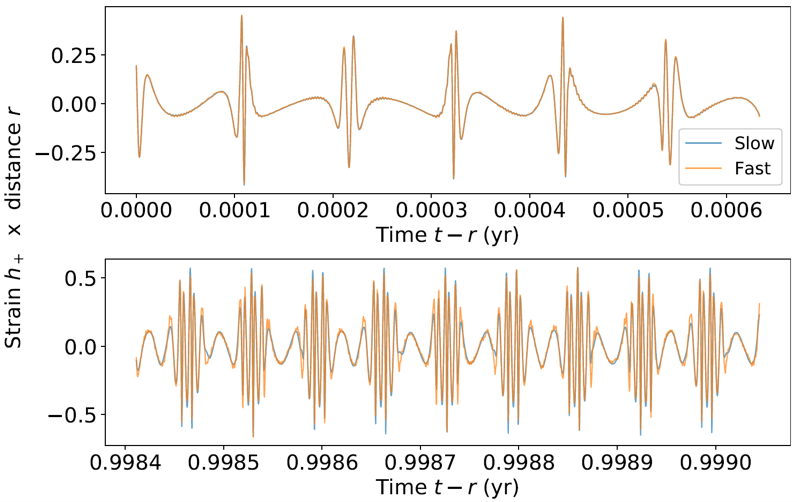
The stellar-mass compact object in an extreme-mass-ratio binary undergoes complex dynamical motion deep within the strong gravitational field of the central massive black hole (Fig 1; illustration credit: M. van de Meent). This complexity is encoded in the gravitational waves emitted by the system, which can be observed with mHz detectors such as LISA (Fig 2; figure credit: A. J. K. Chua et al., PRL 2021).
What are some breakthroughs that astrophysicists hope to attain in the near future?
Seven years on from the first LIGO discovery, our coverage of the gravitational-wave spectrum has been expanded at high frequencies by a growing network of ground-based laser interferometers, and at low frequencies by long-duration pulsar-timing-array projects. The mid-frequency mHz band, where the richest population of sources radiate, remains unprobed. To observe mHz waves with a laser interferometer, we need its arms to be millions of km long, but this far exceeds the Earth’s diameter. For gravitational-wave astronomy, then, space is truly the final frontier (as it is in a certain 1960s vision of the 2260s).
The archetype of a space-based gravitational-wave detector is LISA: an approved ESA–NASA mission that is scheduled for launch in the 2030s. Other similarly ambitious undertakings include the Chinese programs Taiji and Tianqin, along with the Japanese proposal DECIGO. As in the case of Mars exploration, the mere successful execution of such space missions will already comprise significant breakthroughs in not just technology, but also the preparatory science that enables the eventual achievement of their scientific aims. The main science objectives of LISA include: characterising the population of compact stellar binaries in the Milky Way; exploring the life cycle and environment of massive black holes in other galaxies; and probing the fundamental nature of gravity in the strongly gravitating regime.
Many students may be interested in pursuing the field of data science. Share with us how data science is applied in your field of work and its usefulness in general?
Science cannot be performed without data—thus it is not too surprising that the “science” of working with data has itself become an essential part of modern scientific research, especially in the age of big data. However, data science is not always about big data; to highlight an example in gravitational-wave astronomy, the entire data stream from the multi-year LISA mission will fit comfortably on a mid-sized (present-day) flash drive, so the contribution of data science here lies rather in the provision of advanced techniques that are capable of processing, analysing, and drawing reliable inferences from relatively limited data.
My own research straddles the interface between gravitational physics and data science, with a stronger emphasis on the latter area (the majority of theorists in my field lean the other way). Indeed, gravitational-wave astronomy itself is a melting pot of scientific disciplines, with data science as part of an eclectic mix that includes optical metrology, seismology, quantum optics, astrophysics, gravitation, particle physics, and other fields.
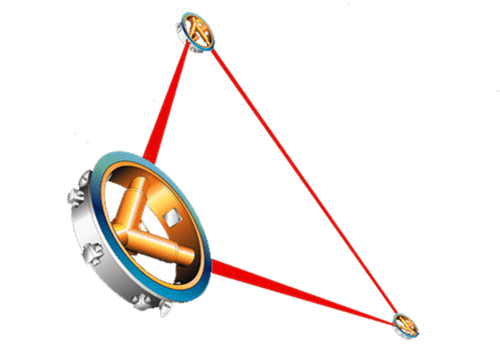
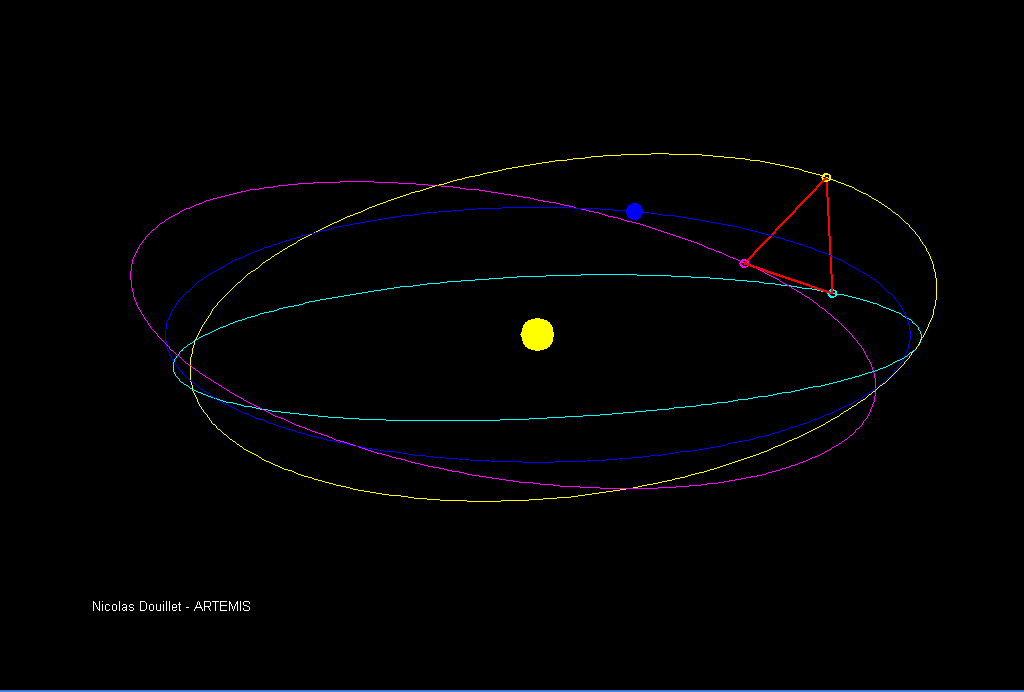
The LISA mission will perform long-baseline laser interferometry using three identical drag-free satellites, each housing two test masses that are effectively in free fall (Fig 3; illustration credit: ESA). These spacecrafts will be separated by 2.5 million km in their stable Earth-trailing orbits around the Sun (Fig 4; animation credit: N. Douillet).
Having been trained in journalism, what motivated your switch to explore the field of astrophysics?
I’ve always liked two languages in particular: English and Mathematics. After completing my science-stream A Levels, one of my (tamer) teenage acts of rebellion was to opt for a journalism major at NTU’s School of Communication and Information. I promptly lost motivation halfway through, but still ended up graduating and working as an editor at the Straits Times for a few years; this was mainly to save up for a second bachelor’s in mathematics and physics, as you can’t easily go from a non-STEM first degree to a higher one in STEM. (The other direction is usually fine, so STEM is clearly the safe bet for anyone on the fence!) My career has since gravitated quite naturally towards astrophysics, which has a sort of universal, evergreen appeal—although I am in truth simply motivated by quantitative problem solving in general, as reflected in the nature of my research rather than its setting.
One lesson that students might take away from this short tale of a rather protracted excursion out of STEM is, I hope, some perspective about choosing their own paths during their late teens or early twenties. It’s important to try and make the right decisions early on, of course—but even if that doesn’t quite happen, I believe it’s still possible to end up exactly where you should be (just takes more time, and a healthy dose of luck).
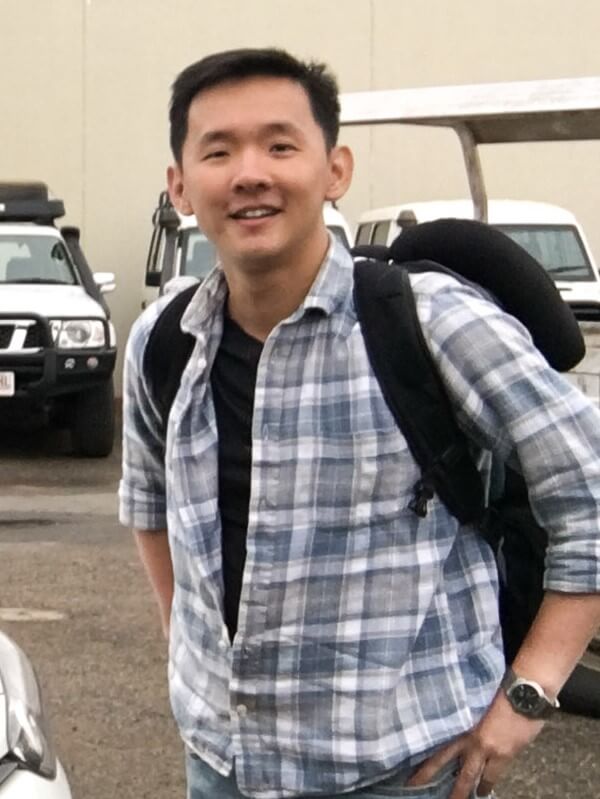