Seeing is Believing: Imaging the chaotic dynamics of the nanoscale world
In his famous lecture “There is plenty of room at the bottom,” Richard Feynman outlined what was to become the blueprint for nanotechnology. In this lecture, he highlighted the role of a focused electron beam which interacts with a matter and can be used for nanoscale probing and sculpting. Fast forward half a century, the ability to visualise and manipulate matter with electrons at the atomic scale resulted in the discovery of new materials and explosive growth of new technologies. For example, today, transmission electron microscopes (TEM) enable imaging and characterisation of materials with an unprecedented atomic resolution, and electron beam lithography is a core technology for patterning nanoscale devices. The reason why TEM can achieve such a high resolution is because the de Broglie wavelength of high energy electrons is in the picometer range, and their resolution for any practical purposes is not diffraction-limited.
However, there is a catch! The problem with fast electrons is that they easily interact with atoms of not only specimens but also air, causing them to undergo undesired scattering and have a very short mean free path. Therefore, the entire column of a TEM has to be kept at a high vacuum. This is usually not a problem for nonvolatile samples, but as we all know, many interesting dynamic physical processes and chemical reactions involve some sort of a liquid or gaseous environment. So the question is then, how can we explore these processes in their relevant environments. Let’s consider how to extend the capabilities of TEM to imaging samples in a liquid environment and note that this can be easily extended into gaseous environments as well.
Our research group has designed a platform that enables imaging in liquids and gases. The core element of this platform is a liquid cell that sandwiches ultrathin solution between two electron transparent SiNx membranes, which isolate the liquid specimen from the vacuum of a TEM.
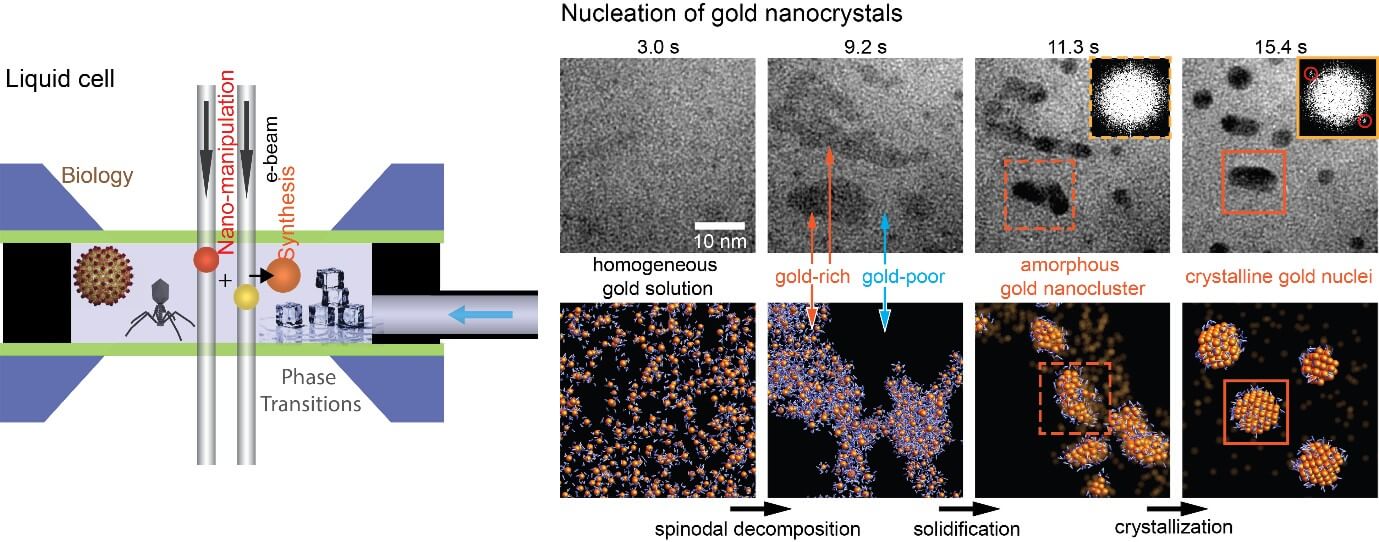
One of the discoveries that we made using this method while imaging the formation of crystals in a solution is a non-classical nucleation pathway. Contrary to classical nucleation theory (CNT), which states that crystals nucleate in a single step, i.e., a crystalline nucleus that can sustain crystal growth forms spontaneously as solute atoms in a solution come together, our observations revealed that crystals nucleate in three steps: 1) the precursor solution phase separates into solute-rich and solute-poor regions, followed by 2) condensation of the solute-rich phase into an amorphous intermediate particle, which then 3) crystalises into a final crystalline nanoparticle. This kind of direct approach to imaging is extremely important because not only it completely changes our understanding of age-long phenomena but also provides a better foundation to design and synthesise new materials.
There are still many challenges when it comes to applying this approach. For example, one of the things that we are trying to do is to image the dynamics of biological molecules with nanometer resolution, which will enable us to identify the role of the structure in their function. However, unlike inorganic materials, most organics and biomolecules are very sensitive to an electron beam and, therefore, damage easily. One way to mitigate this damage is to image with ultralow electron flux, which means that the detectors have to improve significantly, and new image processing toolkits that can de-noise otherwise very noisy images will be needed. Currently, these improvements are being implemented, and moving forward we do remain optimistic that soon it will be possible to catch a glimpse of functioning proteins in their native physiological conditions.
Contributed by Associate Professor Utkur Mirziyodovich, Dean’s Chair